Pediatric immune thrombocytopenia (ITP) treatment
Introduction
Immune thrombocytopenia is the most common acquired bleeding disorder in the pediatric population with an approximate incidence of 5 per 105 children annually (1-3). Despite the overall high disease burden of ITP, many unanswered questions remain about its cause and how to best manage patients.
This review will focus on the initial management of pediatric ITP patients including early laboratory assessments, and front-line therapies. Significant gaps in knowledge have impeded stratifying the best second- and third-line treatment options for patients. Current experience with, mechanisms of action and clinical pearls for using individual drugs will be described. Finally, emerging therapeutics for novel genetic disorders associated with ITP as well as newer adult treatments which may eventually be applied to pediatrics are described.
Initial workup
Upon presentation with isolated thrombocytopenia, in addition to a complete history and exam, the following initial laboratory tests are obligatory: a complete blood count (CBC), reticulocyte count, review of peripheral blood smear. In addition, because they may help guide therapy and uncover common underlying causes of ITP a direct antiglobulin test (DAT) and quantitative immunoglobulin levels are recommended (4). The peripheral smear should be normal with variable platelet size including large platelets and rare giant platelets (Figure 1). A DAT tests for the presence of autoantibodies against erythrocytes. The test is necessary if anti-D immune globulin (anti-D) therapy is being considered because active hemolysis is a contraindication to anti-D use, as will be discussed in detail below. In the absence of hemolysis, a positive DAT in a patient with ITP can provide evidence of more systemic immune dysregulation and warrants investigation of other underlying autoimmune conditions. An ITP patient with a positive DAT in association with hemolytic anemia has Evans syndrome, defined as two autoimmune cytopenias developing concurrently or in succession (5). Identification of Evans syndrome should prompt further evaluation for secondary causes of ITP and has implications for treatment. Some evidence suggests that a positive DAT, in the absence of hemolysis is associated with both evolution to chronic ITP and need for second line treatments (6). Immunoglobulin testing may uncover underlying immune defects which would significantly alter management such as common variable immune deficiency (CVID) or Selective IgA Deficiency. It is valuable to test quantitative immunoglobulins and a DAT prior to initiating therapy. IVIG may obscure low immunoglobulin levels and therefore delay detection of underlying immune deficiencies. Both IVIG and anti-D immune globulin will result in a positive DAT result because of passive antibody transfer.
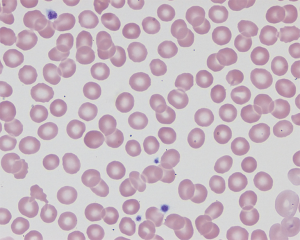
Importance of defining ITP
A diagnosis of exclusion, primary ITP is characterized by isolated thrombocytopenia (platelet count <100×109/L) without another identified disorder leading to immune thrombocytopenia (secondary ITP) (7). ITP can be classified both by the presence or lack of an underlying condition driving thrombocytopenia or by the duration of disease. Newly diagnosed ITP is defined as disease within three months of diagnosis and persistent ITP is disease with a duration between 3 and 12 months. Chronic ITP is defined as thrombocytopenia continuing beyond one year (7).
The ITP disease status and presence of secondary ITP are important factors driving treatment decisions. Causes of secondary ITP are discussed further under second line workup below. For those with longer duration of disease, second line therapies are more likely to be utilized, as quality of life deteriorates more the longer the disease is present (8,9). Refractory or long-standing disease should prompt evaluation for underlying infections, autoimmune or immunodeficiency/immune dysregulation disorders. Certain causes of secondary ITP have specific optimal treatments or contraindications to some therapies.
Treatment strategies
Cautious observation
The majority of children with newly diagnosed ITP are asymptomatic or develop only mild bleeding symptoms. More significant bleeding requiring treatment is more rare, and only 4% of pediatric patients having severe or life threatening bleeding with the incidence of ICH less than 1% (1,4,10). Unlike adult ITP patients, children have fewer comorbid conditions that compound bleeding risk (11). Children with ITP are much more likely than adults to experience spontaneous disease remission, with up to 70% of pediatric patients resolving by 6 months, compared to only 45% of adults (12). Given the lower risk of severe bleeding regardless of platelet count, the likelihood of spontaneous remission, and substantial side effects of ITP therapies, first line management for pediatric ITP is often careful observation. This is contrast to adult ITP patients for whom upfront treatment is considered when the platelet count is below 30×109/L even when asymptomatic or with minor mucocutaneous bleeding (3,4).
Caregivers should be educated on signs of bleeding and counseled on ways to minimize bleeding risk. Anti-platelet agents and anticoagulants must be avoided. Children need to abstain from contact and collision sports and other high-risk activities such as trampoline use. Treatment is indicated if parents do not feel they can appropriately limit their child’s activity, such as in the case of an active toddler or adolescent engaging in sports. Hematology follow up is essential to ensure compliance with activity precautions, monitor for bleeding symptoms, and ensure no other concerning signs or symptoms suggest the need to evaluate for an alternative diagnosis. Psychosocial issues such as compliance concerns or inability to bring the child readily to medical care must be accounted for when deciding to observe. If appropriate return to medical care cannot be guaranteed, the patient should be treated.
Children with severe or emergent bleeding such as hematuria, hematochezia, or other internal hemorrhage such as intracranial bleeding require immediate and often multi-modal treatment. Those with mucosal bleeding (such as wet purpura, epistaxis, and menorrhagia) (Figure 2) have increased risk for severe bleeding and in this scenario, treatment is indicated to rapidly achieve a hemostatic platelet count.
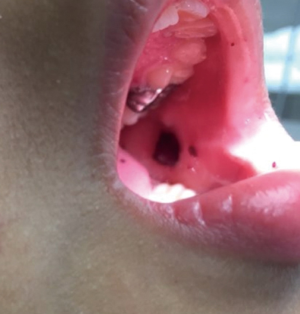
Ultimately, the decision to treat pediatric ITP patients is an individualized one and shared decision making between the patient, caregiver and physician is critical.
For children requiring therapy but without life threatening bleeding, corticosteroids are the recommended first line therapy over IVIG or anti-D (3). Corticosteroids are suggested over IVIG or anti-D by the 2019 American Society of Hematology ITP guidelines, due to low cost, universal availability, ease of outpatient administration, no exposure to multiple blood donors and overall minimal side effects associated with short courses of steroids (3). Importantly, severe life threatening side effects are reported for IVIG and anti-D. As described below, anti-D has a black box warning for intravascular hemolysis (13) and IVIG carries a black box warning for both renal failure and thrombosis (14,15). Studies on the effectiveness of steroids and formal trials comparing first line agents are lacking (3). Because oral steroids have a slower time to effect, IV steroids, IVIG or anti-D are more appropriate when a rapid rise in platelets is needed in the setting of severe mucosal bleeding. Description of the three front line medications for ITP is found in Table 1.
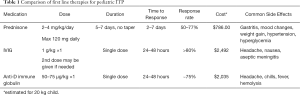
Full table
Corticosteroids
Several corticosteroid regimens are utilized in practice, but have not been directly compared. Guidelines from the American Society of Hematology recommend a 5–7 day course of prednisone dosed at 2–4 mg/kg/day (3). Seventy-five percent of children respond to steroids, with platelets recovering to hemostatic range by 2–7 days (16) (Table 1). If a more rapid rise in platelets is desired, IV methylprednisolone may be used. Studies comparing outcomes between anti-D versus methylprednisolone (17) and comparing methylprednisolone with dexamethasone (18) showed similar response rates with minor side effects in all groups.
Mechanism
There are multiple molecular mechanisms of action for corticosteroids in ITP. Steroids may increase platelet production (19), decrease autoantibody production (20), decrease platelet clearance (21), and reduce structural changes at the endothelium thereby decreasing bleeding and platelet consumption (22).
Risks and adverse events
There are substantial side effects of corticosteroid therapies, especially if used for a prolonged duration at moderate to high doses. Common side effects include weight gain, mood changes, sleep disturbance, gastritis, GI bleeding, hypertension, hyperglycemia, and when used chronically, cataracts and osteoporosis. As an immune suppressive medication, steroids should not be used in the setting of severe infection and can specifically result in re-activation of varicella. Given the substantial adverse effects of corticosteroids, they should be limited to as brief a course as possible, especially in children. If no response is seen, alternative therapies should be pursued (3).
Intravenous immune globulin
Intravenous immune globulin (IVIG) is a commonly used agent for upfront treatment of pediatric ITP. The use of this therapy in children is specifically discussed in detail elsewhere in this issue.
Anti-D immune globulin (Rho(D) immune globulin, anti-D)
Anti-D is another frontline option in children who require treatment. Anti-D has advantages as a rapid IV infusion with lower donor exposure than IVIG. Anti-D typically induces a rise in platelet counts within 24–48 hours for approximately 50–77% of children (4,23).
Mechanism
While the mechanism is not fully understood, it is postulated that anti-D antibodies coat circulating erythrocytes, which then travel to the spleen. Opsonized red cells saturate Fc receptors on reticuloendothelial cells. This allows antibody coated platelets to evade binding by the spleen thereby increasing their survival in circulation (24). Because anti-D requires binding to Rh-antigens on erythrocytes, it is only effective for individuals who are Rh positive. Additionally, anti-D is generally ineffective in asplenic patients.
Risks and adverse events
The use of anti-D has decreased since it was first approved for use in ITP in 1995. A major contributor to the decreased utilization of anti-D was the FDA black box warning focused on severe intravascular hemolysis issued in 2010 (13). Though rare, anti-D’s most feared side effect is intravascular hemolysis leading to severe anemia, multi-organ failure, acute respiratory distress syndrome and death (13). Although these life threatening events occurred in predisposed patients (25), the FDA further advises close monitoring for 8 hours post-infusion with periodic urine assessment looking for evidence of hemolysis. Supportive care is recommended for individuals who experience severe intravascular hemolysis which may include transfusions or respiratory support. Because anti-D binds erythrocytes and targets them for destruction by the reticuloendothelial system, some degree of hemolysis is inherent with anti-D use. The average decrease in hemoglobin following anti-D use is 1.7 g/dL (23). Other side effects are less severe and include fevers, chills and headache occurring in 1–2% of patients (26).
Due to the expected hemolysis, children should be screened for pre-existing hemolysis or risk factors prior to receiving anti-D. A DAT is a recommended component of the initial laboratory evaluation of ITP patients both to determine safety and may also have clinical implications (4,6). Administration should be avoided in children with abnormal renal function and due to risk of inducing a severe reaction, anti-D should not be given in known or suspected EBV infection, or highly febrile children (27).
Emergency care
Regardless of disease status (new, persistent, or chronic), for severe uncontrolled bleeding, multiple treatment modalities may be applied together including platelet transfusion, IV steroids, IVIG and/or anti-D. If bleeding does not improve rapidly and the patient continues to have major bleeding, emergency splenectomy may be the only option.
While platelet transfusion is usually avoided because transfused platelets are thought to be rapidly consumed in the setting of ITP, some patients may have a modest increase in platelets and have reduction in bleeding (28,29). Individuals who have a response to platelet transfusions may represent a distinct ITP phenotype that is T-cell mediated as opposed to antibody-driven (30). Front-line therapies given in concert with transfusions may prolong the life-span of transfused platelets (16,29).
Increasingly, thrombopoietin receptor agonists (TPO-RAs) are being used earlier in the management of pediatric ITP. TPO-RAs may have a role as adjunct in the setting of severe or life-threatening bleeding. While these agents do not generate a rapid platelet count increase, they may help sustain platelet counts after the effect of initial IV therapies wane. In the case of ICH, medical management is initiated immediately and emergency splenectomy and/or neurosurgical intervention considered.
Secondary ITP diagnostic workup
Children with chronic ITP warrant further evaluation for secondary ITP, or underlying conditions that lead to immune thrombocytopenia. Similar to those with long standing single cytopenias, children with multi-lineage autoimmune cytopenias require more extensive testing. Evans syndrome, defined as two or more cytopenias, either occurring concurrently or sequentially (31), is most often characterized by ITP and autoimmune hemolytic anemia (AIHA) (32). Evans syndrome was previously defined as idiopathic, but increasingly underlying causative lesions are being identified, including novel genetic syndromes (33).
Conditions associated with secondary ITP include lymphoproliferative disorders [e.g., autoimmune lymphoproliferative syndrome (ALPS)], immunodeficiency syndromes (e.g., CVID, DiGeorge syndrome, SCID), rheumatologic conditions (e.g., Systemic lupus erythematosus (SLE), Antiphospholipid antibody syndrome, Sjögren syndrome, juvenile rheumatoid arthritis), malignancies (e.g., Hodgkin lymphoma, non-Hodgkin lymphoma), or chronic infections (e.g., HIV, hepatitis, cytomegalovirus, H. Pylori). In the setting of chronic infections, priority should be given to treating the underlying condition, which in itself, may ameliorate thrombocytopenia (34). For example, HIV should be managed with antiretrovirals, and if ITP requires treatment, IVIG, steroids or anti-D can be used. In the setting of Hepatitis C virus infection, treatment involves antivirals and interferon. Interferon may drop platelet counts, and IVIG is preferentially used to treat thrombocytopenia in this setting, as steroids may increase viral loads (34).
Work-up is tailored based on the child’s history, individual risk factors, physical exam findings, and family history. Minimum screening for disorders frequently implicated as causing secondary ITP or those for which diagnosis alters management substantially are outlined in Table 2. While a bone marrow evaluation is not recommended for new pediatric ITP patients and is not required prior to starting therapy, in the setting of chronic, refractory disease, it may be considered (3).
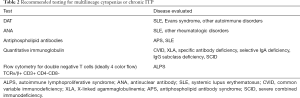
Full table
Second line therapy options
Second line agents are indicated for children who do not respond or relapse after first line agents. These first line therapies are utilized with the goal of rapidly increasing the platelet count, thereby mitigating severe bleeding risk, but are not intended for use as a maintenance therapy, or to elicit a durable response in those with persistent or chronic ITP. Second line therapies in children are similar to those used in adults, however splenectomy is avoided in children given the likelihood of spontaneous resolution in pediatric populations and potential for complications. Splenectomy is generally only considered after failure of medical management including combination therapy in a child over age 5 with ITP for at least 12 months, or in the setting of uncontrolled life-threatening bleeding.
TPO-RAs: eltrombopag and romiplostim
Increasingly TPO-RAs are being utilized as the initial second line agent for pediatric ITP patients who do not respond to upfront therapies (corticosteroids, IVIG or anti-D). The most recent American Society of Hematology guidelines, published in 2019, suggest the use of TPO-RAs over rituximab and splenectomy in children (3). In contrast, selection of TPO-RAs versus rituximab or splenectomy in adults depends more heavily on patient preferences regarding use of daily medications and feelings about surgical interventions (3).
Eltrombopag was approved for use in children with chronic ITP in 2015 following two multicenter, double-blind, placebo controlled trials (PETIT and PETIT2) demonstrated its efficacy at raising the platelet count with a favorable side effect profile (35,36). Romiplostim was approved in December 2018 for use in children over 1 year of age who had refractory disease persisting beyond 6 months, however it had been used off-label for many years prior to this (37). The majority of children who received eltrombopag or romiplostim in clinical trials had a favorable response to the drugs (35,36,38-40).
Selecting eltrombopag or romiplostim depends on the preferences of the patient, their family and comfort of the provider with prescribing one or the other. Differences in administration heavily impact choice of TPO-RAs. Eltrombopag is dosed once daily and is available as an oral tablet or as a powder for suspension (41). A more challenging issue with eltrombopag administration in the pediatric population is the need to space doses 2 hours before or 4 hours after dairy consumption, as divalent cations such as calcium decrease its absorption (42). Romiplostim is dosed as a weekly subcutaneous injection. Romiplostim is not FDA approved for home administration, so families may be required to make weekly clinic visits to receive treatment. If patients are transitioned between one TPO-RA to the other, the likelihood of a response with an alternate agent is approximately 75% (43).
Mechanism
Both eltrombopag and romiplostim act by binding the TPO receptor, c-mpl, thereby driving increased platelet production by megakaryocytes. Structurally homologous to endogenous TPO, first generation TPO-RAs, formulated as pegylated recombinant human megakaryocyte growth and development factor (PEG-rHuMGDF) and recombinant human thrombopoietin (rhTPO), induced antibody development against endogenous TPO (44-46). However, second generation TPO-RAs lack sequence homology with endogenous TPO, bind different regions of the TPO receptor, and are less immunogenic (47). Aside from stimulating platelet production, there is also some evidence to suggest that TPO-RAs have immunomodulatory effects (48,49). This is being studies currently in pediatric ITP populations (NCT03939637).
Romiplostim is comprised of two peptides conjugated to the IgG1 heavy chain and binds the extracellular binding domain of c-mpl. Eltrombopag, is a small, non-peptide molecule which interacts with the juxtamembrane domain of the TPO receptor (Figure 3). Both romiplostim and eltrombopag stimulate platelet production via multiple signaling pathways including the SHC-Ras-Raf pathway, JAK-STAT pathway and PI3k-Akt signaling (50).
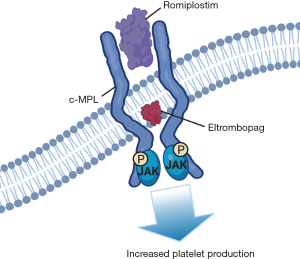
Risks and adverse effects
Overall, the TPO-RAs are well tolerated by pediatric patients and shown to be safe in clinical trials.
Thromboembolic events are a concern in ITP patients treated with TPO-RAs, but recent data show the risk does not appear to be elevated compared to children with ITP who were treated with other therapies (50). Concern for thrombosis also draws largely on experience in adults, who have other comorbidities contributing to thrombosis risk (50). Pediatric patients have not developed thrombi while on TPO-RAs in clinical trials, but theoretically, adolescents with additional risk factors such as obesity or estrogen contraceptive use may have a risk more similar to adult ITP patients (38,51).
Based on lens changes in animal models, cataract development was an initial concern with TPO-RA use. In the PETIT2 trial, 1 patient had progression of an existing cataract and another developed a new cataract, however both patients were also treated with steroids (36).
By stimulating megakaryocytes, which then release cytokines that promote collagen synthesis by marrow fibroblasts, both eltrombopag and romiplostim can lead to reticulin fiber deposition in the bone marrow (52,53). However, in studies of both agents, bone marrow fibrosis was extremely rare, and when it did develop, was mild and did not impact peripheral blood counts. Fibrosis also appears to be reversible on discontinuation (54,55).
Eltrombopag has hepatic metabolism and drug levels may be up to 41% higher in the setting of even mild hepatic dysfunction (56). Eltrombopag can cause transaminitis, however this is typically mild (36,39). Finally, as a chelator, eltrombopag can also result in decreased iron absorption and lead to iron deficiency (35,57).
Because divalent cations, such as calcium, decrease eltrombopag absorption, it must be taken on an empty stomach and doses should be timed at least 4 hours before or after dairy and other calcium rich food consumption (42). This factor significantly limits the use of eltrombopag in children.
Rituximab
Though not FDA approved for use in ITP, based on experience with other autoimmune conditions, rituximab has long been used for patients with ITP and inadequate response to upfront therapy. Dosing in ITP is typically 375 mg/m2 in 4 weekly infusions (58). While TPO-RAs have around 70–80% response rate (35,36,59), reported response rates to rituximab are approximately 60% (60). Response to rituximab is frequently not sustained, with reports showing only 26% of children maintain their platelet counts at 5 years from rituximab dosing (60).
Ascertaining the underlying cause of secondary ITP is critical when planning to use rituximab for refractory disease. In those with SLE, rituximab may have better response rates than primary ITP patients (61). However, there are conditions where rituximab should be avoided. In ALPS, those who receive rituximab are at increased risk of having prolonged B cells depletion and hypogammaglobulinemia. Prolonged neutropenia is reported in ALPS patients and those with underling immunodeficiencies treated with rituximab (33,62). In general, we recommend all children receiving rituximab have immunoglobulin levels tested and be screened for normal B cell populations as a baseline prior to dosing. Some patients who receive rituximab experience symptomatic hypogammaglobulinemia and increased infection risk (63). Patients with underlying immune defects such as CVID are at particular risk for this outcome (63).
Mechanism
Rituximab is a chimeric monoclonal antibody directed against CD20, expressed on B cells (64). Once bound, rituximab triggers B cell destruction via complement or Fc-receptor mediated clearance (65). With depleted B cells, the production of auto-antibodies directed against platelet glycoproteins is reduced.
Risks and adverse effects
The most common adverse events associated with rituximab are related to infusion reactions including fevers, myalgias, hives, chest tightness, headache and hypertension. Severe side effects are exceedingly rare in children with ITP and include serum sickness or progressive multifocal leukoencephalopathy (66). Finally, rituximab can reactivate hepatitis B infection resulting in fulminant, even fatal disease (66).
Splenectomy
In the past, splenectomy was a commonly utilized second line treatment, but is now rarely performed (67,68). When used, it should be reserved for children with significant bleeding, over the age of 5, who have tried and failed available medical management, including combination therapies. Unless being used for emergency bleeding control, an invasive and permanent intervention such as splenectomy should also be limited to those with chronic ITP due to the high likelihood of spontaneous disease resolution (3). Prior to surgery, vaccinations should be up to date including Haemophilus influenza type B, meningococcal and pneumococcal 13-valent conjugate vaccines, followed by pneumococcal 23-valent vaccine (3).
Pediatric response rates to splenectomy are around 60-70% within a day of surgery, hence its application in the case of life-threatening bleeding. Over 4 years, 80% of splenectomized children maintain a response (16).
Risks and adverse effects
Splenectomy results in an increased risk for infection, both in the immediate post-operative period as well as an overall life-long increased risk for sepsis or invasive infection. There is increased risk for deep venous thrombus and pulmonary hypertension development as well, though most reports on these outcomes focus on adults. In addition, there is mortality and morbidity risk associated with the surgery itself (69).
Alternative treatments
After first- and second-line therapies have been tried, the next therapy option is selected in discussions between the medical team, caregivers and patient. In a study focused on treatment choices from the ITP Consortium of North America, the most common reason to select a particular agent was the possibility of long-term remission, followed by parental or patient preference, and side effect profile (68). Other factors which impact decision making include the provider’s experience and ease of administration for the individual drugs (33).
There is a paucity of large, formal studies evaluating outcomes of third line agents in pediatric ITP and certainly, there are no randomized controlled trials comparing the many available therapies directly. Other agents used as third line management include purine analogues (azathioprine, mercaptopurine), mycophenolate mofetil, sirolimus, cyclophosphamide, cyclosporine A, dapsone, danazol and vincristine. Collectively, initial response rates for these drugs range from around 30–60% (3).
For refractory patients, combination therapies may also be trialed. In our experience, using medications which target different disease mechanisms is more efficacious.
Emerging therapies
Targeted therapies
The genetic and molecular understanding of ITP pathogenesis is expanding. Increasingly, patients thought to have primary ITP without a typical response to therapies or an unexpected disease course, are found to carry novel genetic alterations resulting in loss of immune tolerance. Armed with this knowledge and increasing availability of genetic testing, an individual patient’s treatment regimen can be refined to their specific condition, rather than trialing a series of broad immune suppressive agents.
CTLA- 4 haploinsufficiency and LRBA deficiency
Cytotoxic T-lymphocyte-associated protein 4 (CTLA-4) is constitutively expressed on regulatory T cells and inhibits T cell activation (70,71). LPS-responsive beige-like receptor anchor protein (LRBA) is thought to be a regulator of CTLA-4 function. Impacting the same molecular signaling pathway, both CTLA-4 haploinsufficiency and LRBA deficiency lead to abnormal activation and proliferation of T cells resulting in loss of immune tolerance (72-75). While patients have other features including recurrent infections, hypogammaglobulinemia, and lymphocytic infiltration of multiple organ systems, ITP is a feature of both conditions (70,76,77). Abatacept, a fusion protein comprised of the extracellular domain of CTLA-4 bound to IgG1, restores CTLA-4 and ameliorates symptoms of autoimmunity (76,78).
Both these conditions present in the early adolescent population and are an important consideration for chronic ITP patients with additional features of immune dysregulation (74).
PI3Kδ syndrome
Gain-of-function mutations in PI3Kδ lead to activation of the mTOR pathway and, though Akt phosphorylation, may promote effector T cell development (79). Presenting in children age 1 to 7 years of age, those with PI3Kδ syndrome have autoimmune cytopenias, lymphadenopathy, hepatosplenomegaly and immunodeficiency (80). Sirolimus is an mTOR inhibitor and has been used in treatment of PI3Kδ syndrome. A selective PI3Kδ inhibitor, Leniolisib is currently in clinical trials (81).
STAT1 and 3 gain- of- function
Excess STAT1 signaling results in skewed Th17 differentiation and hyperresponsiveness to IFN-γ (33). Clinically, patients experience autoimmunity and chronic mucocutaneous candidiasis (82). When stimulated, Janus kinase (JAK) recruit STATs, leading to signal transduction. Ruxolitinib, a JAK inhibitor, acts to correct the molecular defect in these patients, though STAT levels may not correlate directly to symptomatology (83). Excess STAT3 activation leads to suppressed apoptosis via cytokine signaling, including IL-6 (84). Tocilizumab, an IL-6 inhibitor and ABT-737, which targets anti-apoptotic protein Bcl2, are being used as treatments for this condition (85).
Potential future pediatric therapies
A number of newer agents are being employed for adult ITP management which may eventually have utility in pediatric populations.
A transcytosis receptor, the neonatal Fc receptor (FcRn) regulates circulating IgG (86). Inhibition of the FcRn, results in increased lysosomal IgG degradation and subsequently decreases serum IgG levels. Efgardigomod and rozanolixizumab are both novel FcRn receptor antagonists. Phase 2 trials of efgardigomod, a weekly intravenous infusion, showed efficacy and favorable side effect profiles in adults with refractory ITP (87). Rozanolixizumab, a subcutaneous injection, raised platelet counts and decreased IgG levels with minimal side effects in phase 2 trials of adult ITP patients with multiply refractory disease (88). It is currently in phase 3 trials for adult ITP patients (NCT04200456). Neither FcRn antagonist has been studied in children.
Avatrombopag is an oral TPO-RA first approved in adults with ITP and chronic liver disease. As of June 2019, its approval was expanded to use in adults with chronic ITP who failed prior therapies (89). Avatrombopag is attractive as a potential future option in children because it is oral and does not have the same dietary restrictions that accompany eltrombopag (90). At the time this article was being written, avatrombopag was going into pediatric clinical trials (NCT04516967).
Fostamatinib is a spleen tyrosine kinase (SYK) inhibitor which inhibits Fc receptor mediated platelet destruction. It was approved in 2018 for use in refractory adult ITP patients. A phase III study in multiply refractory adult chronic ITP patients demonstrated 43% of patients achieved a platelet count of ≥50,000/µL at 3 months (91). Due to concerns on effects on cartilage in growing children, it has yet to be studied in pediatrics.
Conclusion
For many children ITP symptoms are mild and self-resolve, and it would initially seem that treatment is straight forward. However, pediatric ITP is a heterogeneous disorder, with each patient’s case differing in bleeding phenotype, duration of disease and response to therapy. In particular, for those with chronic ITP, multi-lineage cytopenias or individuals with thrombocytopenia which is a component of another underlying systemic disorder, management is even more complex. The field of ITP study continues to identify more genetic risk factors. These can now be leveraged to both elucidate the cause of an individual child’s ITP, and be used to develop better targeted therapies in the future.
Acknowledgments
Funding: None.
Footnote
Provenance and Peer Review: This article was commissioned by the Guest Editors (John W. Semple and Rick Kapur) for the series “Treatment of Immune Thrombocytopenia (ITP)” published in Annals of Blood. The article was sent for external peer review organized by the Guest Editors and the editorial office.
Peer Review File: Available at http://dx.doi.org/10.21037/aob-20-96
Conflicts of Interest: Both authors have completed the ICMJE uniform disclosure form (available at http://dx.doi.org/10.21037/aob-20-96). The series “Treatment of Immune Thrombocytopenia (ITP)” was commissioned by the editorial office without any funding or sponsorship. Dr. JMD reports grants and personal fees from Novartis, personal fees from Dova, personal fees from Amgen, personal fees from Uptodate, outside the submitted work. The authors have no other conflicts of interest to declare.
Ethical Statement: The authors are accountable for all aspects of the work in ensuring that questions related to the accuracy or integrity of any part of the work are appropriately investigated and resolved.
Open Access Statement: This is an Open Access article distributed in accordance with the Creative Commons Attribution-NonCommercial-NoDerivs 4.0 International License (CC BY-NC-ND 4.0), which permits the non-commercial replication and distribution of the article with the strict proviso that no changes or edits are made and the original work is properly cited (including links to both the formal publication through the relevant DOI and the license). See: https://creativecommons.org/licenses/by-nc-nd/4.0/.
References
- Terrell DR, Beebe LA, Vesely SK, et al. The incidence of immune thrombocytopenic purpura in children and adults: A critical review of published reports. Am J Hematol 2010;85:174-80. [Crossref] [PubMed]
- Neunert CE, Buchanan GR, Imbach P, et al. Severe hemorrhage in children with newly diagnosed immune thrombocytopenic purpura. Blood 2008;112:4003-8. [Crossref] [PubMed]
- Neunert C, Terrell DR, Arnold DM, et al. American Society of Hematology 2019 guidelines for immune thrombocytopenia. Blood Adv 2019;3:3829-66. [Crossref] [PubMed]
- Provan D, Arnold DM, Bussel JB, et al. Updated international consensus report on the investigation and management of primary immune thrombocytopenia. Blood Adv 2019;3:3780-817. [Crossref] [PubMed]
- Miano M. How I manage Evans Syndrome and AIHA cases in children. Br J Haematol 2016;172:524-34. [Crossref] [PubMed]
- Kim TO, Grimes AB, Kirk S, et al. Association of a positive direct antiglobulin test with chronic immune thrombocytopenia and use of second line therapies in children: A multi-institutional review. Am J Hematol 2019;94:461-6. [Crossref] [PubMed]
- Rodeghiero F, Stasi R, Gernsheimer T, et al. Standardization of terminology, definitions and outcome criteria in immune thrombocytopenic purpura of adults and children: report from an international working group. Blood 2009;113:2386-93. [Crossref] [PubMed]
- Neunert CE, Buchanan GR, Blanchette V, et al. Relationships among bleeding severity, health-related quality of life, and platelet count in children with immune thrombocytopenic purpura. Pediatr Blood Cancer 2009;53:652-4. [Crossref] [PubMed]
- Grace RF, Shimano KA, Bhat R, et al. Second-line treatments in children with immune thrombocytopenia: Effect on platelet count and patient-centered outcomes. Am J Hematol 2019;94:741-50. [Crossref] [PubMed]
- Neunert C, Noroozi N, Norman G, et al. Severe bleeding events in adults and children with primary immune thrombocytopenia: a systematic review. J Thromb Haemost 2015;13:457-64. [Crossref] [PubMed]
- Despotovic JM, Grimes AB. Pediatric ITP: is it different from adult ITP? Hematology Am Soc Hematol Educ Program 2018;2018:405-11. [Crossref] [PubMed]
- Schifferli A, Holbro A, Chitlur M, et al. A comparative prospective observational study of children and adults with immune thrombocytopenia: 2-year follow-up. Am J Hematol 2018;93:751-9. [Crossref] [PubMed]
- Administration FaD. Package Insert- WinRho SDF. 2019.
- Mitka M. FDA: Thrombosis risk with immune globulin products. JAMA 2013;310:247. [Crossref] [PubMed]
- Administration FaD. Gamunex-C Package Insert. 2015.
- Provan D, Stasi R, Newland AC, et al. International consensus report on the investigation and management of primary immune thrombocytopenia. Blood 2010;115:168-86. [Crossref] [PubMed]
- Celik M, Bulbul A, Aydogan G, et al. Comparison of anti-D immunoglobulin, methylprednisolone, or intravenous immunoglobulin therapy in newly diagnosed pediatric immune thrombocytopenic purpura. J Thromb Thrombolysis 2013;35:228-33. [Crossref] [PubMed]
- Su Y, Xu H, Xu Y, et al. A retrospective analysis of therapeutic responses to two distinct corticosteroids in 259 children with acute primary idiopathic thrombocytopenic purpura. Hematology 2009;14:286-9. [Crossref] [PubMed]
- Gernsheimer T, Stratton J, Ballem PJ, et al. Mechanisms of response to treatment in autoimmune thrombocytopenic purpura. N Engl J Med 1989;320:974-80. [Crossref] [PubMed]
- Mizutani H, Furubayashi T, Imai Y, et al. Mechanisms of corticosteroid action in immune thrombocytopenic purpura (ITP): experimental studies using ITP-prone mice, (NZW x BXSB) F1. Blood 1992;79:942-7. [Crossref] [PubMed]
- Crow AR, Lazarus AH. Role of Fcgamma receptors in the pathogenesis and treatment of idiopathic thrombocytopenic purpura. J Pediatr Hematol Oncol 2003;25:S14-8. [Crossref] [PubMed]
- Kitchens CS, Pendergast JF. Human thrombocytopenia is associated with structural abnormalities of the endothelium that are ameliorated by glucocorticosteroid administration. Blood 1986;67:203-6. [Crossref] [PubMed]
- Long M, Kalish LA, Neufeld EJ, et al. Trends in anti-D immune globulin for childhood immune thrombocytopenia: usage, response rates, and adverse effects. Am J Hematol 2012;87:315-7. [Crossref] [PubMed]
- Ware RE, Zimmerman SA. Anti-D: mechanisms of action. Semin Hematol 1998;35:14-22. [PubMed]
- Despotovic JM, Lambert MP, Herman JH, et al. RhIG for the treatment of immune thrombocytopenia: consensus and controversy (CME). Transfusion 2012;52:1126-36; quiz 5. [Crossref] [PubMed]
- Freiberg A, Mauger D. Efficacy, safety, and dose response of intravenous anti-D immune globulin (WinRho SDF) for the treatment of idiopathic thrombocytopenic purpura in children. Semin Hematol 1998;35:23-7. [PubMed]
- Levendoglu-Tugal O, Jayabose S. Intravenous anti-D immune globulin-induced intravascular hemolysis in Epstein-Barr virus-related thrombocytopenia. J Pediatr Hematol Oncol 2001;23:460-3. [Crossref] [PubMed]
- Carr JM, Kruskall MS, Kaye JA, et al. Efficacy of platelet transfusions in immune thrombocytopenia. Am J Med 1986;80:1051-4. [Crossref] [PubMed]
- Spahr JE, Rodgers GM. Treatment of immune-mediated thrombocytopenia purpura with concurrent intravenous immunoglobulin and platelet transfusion: a retrospective review of 40 patients. Am J Hematol 2008;83:122-5. [Crossref] [PubMed]
- Guo L, Yang L, Speck ER, et al. Allogeneic platelet transfusions prevent murine T-cell-mediated immune thrombocytopenia. Blood 2014;123:422-7. [Crossref] [PubMed]
- Evans RS, Takahashi K, Duane RT, et al. Primary thrombocytopenic purpura and acquired hemolytic anemia; evidence for a common etiology. AMA Arch Intern Med 1951;87:48-65. [Crossref] [PubMed]
- Norton A, Roberts I. Management of Evans syndrome. Br J Haematol 2006;132:125-37. [Crossref] [PubMed]
- Kim TO, Despotovic JM. Primary and Secondary Immune Cytopenias: Evaluation and Treatment Approach in Children. Hematol Oncol Clin North Am 2019;33:489-506. [Crossref] [PubMed]
- Neunert C, Lim W, Crowther M, et al. The American Society of Hematology 2011 evidence-based practice guideline for immune thrombocytopenia. Blood 2011;117:4190-207. [Crossref] [PubMed]
- Bussel JB, de Miguel PG, Despotovic JM, et al. Eltrombopag for the treatment of children with persistent and chronic immune thrombocytopenia (PETIT): a randomised, multicentre, placebo-controlled study. Lancet Haematol 2015;2:e315-25. [Crossref] [PubMed]
- Grainger JD, Locatelli F, Chotsampancharoen T, et al. Eltrombopag for children with chronic immune thrombocytopenia (PETIT2): a randomised, multicentre, placebo-controlled trial. Lancet 2015;386:1649-58. [Crossref] [PubMed]
- Neunert CE, Rose MJ. Romiplostim for the management of pediatric immune thrombocytopenia: drug development and current practice. Blood Adv 2019;3:1907-15. [Crossref] [PubMed]
- Tarantino MD, Bussel JB, Blanchette VS, et al. Long-term treatment with romiplostim and treatment-free platelet responses in children with chronic immune thrombocytopenia. Haematologica 2019;104:2283-91. [Crossref] [PubMed]
- Bussel JB, Buchanan GR, Nugent DJ, et al. A randomized, double-blind study of romiplostim to determine its safety and efficacy in children with immune thrombocytopenia. Blood 2011;118:28-36. [Crossref] [PubMed]
- Mathias SD, Li X, Eisen M, et al. A Phase 3, Randomized, Double-Blind, Placebo-Controlled Study to Determine the Effect of Romiplostim on Health-Related Quality of Life in Children with Primary Immune Thrombocytopenia and Associated Burden in Their Parents. Pediatr Blood Cancer 2016;63:1232-7. [Crossref] [PubMed]
- Novartis. Promacta Package Insert. 2020.
- Kim TO, Despotovic J, Lambert MP. Eltrombopag for use in children with immune thrombocytopenia. Blood Adv 2018;2:454-61. [Crossref] [PubMed]
- González KJ, Zuluaga SO, DaRos CV, et al. Sequential treatment with thrombopoietin-receptor agonists (TPO-RAs) in immune thrombocytopenia (ITP): experience in our center. Ann Hematol 2017;96:507-8. [Crossref] [PubMed]
- Kuter DJ. Whatever happened to thrombopoietin? Transfusion 2002;42:279-83. [Crossref] [PubMed]
- Li J, Yang C, Xia Y, et al. Thrombocytopenia caused by the development of antibodies to thrombopoietin. Blood 2001;98:3241-8. [Crossref] [PubMed]
- Wang S, Yang R, Zou P, et al. A multicenter randomized controlled trial of recombinant human thrombopoietin treatment in patients with primary immune thrombocytopenia. Int J Hematol 2012;96:222-8. [Crossref] [PubMed]
- Kuter DJ. The biology of thrombopoietin and thrombopoietin receptor agonists. Int J Hematol 2013;98:10-23. [Crossref] [PubMed]
- Townsley DM, Scheinberg P, Winkler T, et al. Eltrombopag Added to Standard Immunosuppression for Aplastic Anemia. N Engl J Med 2017;376:1540-50. [Crossref] [PubMed]
- González-López TJ, Pascual C, Alvarez-Roman MT, et al. Successful discontinuation of eltrombopag after complete remission in patients with primary immune thrombocytopenia. Am J Hematol 2015;90:E40-3. [Crossref] [PubMed]
- Gilbert MM, Grimes AB, Kim TO, et al. Romiplostim for the Treatment of Immune Thrombocytopenia: Spotlight on Patient Acceptability and Ease of Use. Patient Prefer Adherence 2020;14:1237-50. [Crossref] [PubMed]
- Neunert C, Despotovic J, Haley K, et al. Thrombopoietin Receptor Agonist Use in Children: Data From the Pediatric ITP Consortium of North America ICON2 Study. Pediatr Blood Cancer 2016;63:1407-13. [Crossref] [PubMed]
- Cuker A, Chiang EY, Cines DB. Safety of the thrombopoiesis-stimulating agents for the treatment of immune thrombocytopenia. Curr Drug Saf 2010;5:171-81. [Crossref] [PubMed]
- Kuter DJ, Mufti GJ, Bain BJ, et al. Evaluation of bone marrow reticulin formation in chronic immune thrombocytopenia patients treated with romiplostim. Blood 2009;114:3748-56. [Crossref] [PubMed]
- Rodeghiero F, Stasi R, Giagounidis A, et al. Long-term safety and tolerability of romiplostim in patients with primary immune thrombocytopenia: a pooled analysis of 13 clinical trials. Eur J Haematol 2013;91:423-36. [Crossref] [PubMed]
- Wong RSM, Saleh MN, Khelif A, et al. Safety and efficacy of long-term treatment of chronic/persistent ITP with eltrombopag: final results of the EXTEND study. Blood 2017;130:2527-36. [Crossref] [PubMed]
- Burness CB, Keating GM, Garnock-Jones KP. Eltrombopag: A Review in Paediatric Chronic Immune Thrombocytopenia. Drugs 2016;76:869-78. [Crossref] [PubMed]
- Vlachodimitropoulou E, Chen YL, Garbowski M, et al. Eltrombopag: a powerful chelator of cellular or extracellular iron(III) alone or combined with a second chelator. Blood 2017;130:1923-33. [Crossref] [PubMed]
- Cuker A. Transitioning patients with immune thrombocytopenia to second-line therapy: Challenges and best practices. Am J Hematol 2018;93:816-23. [Crossref] [PubMed]
- Cuker A, Neunert CE. How I treat refractory immune thrombocytopenia. Blood 2016;128:1547-54. [Crossref] [PubMed]
- Patel VL, Mahevas M, Lee SY, et al. Outcomes 5 years after response to rituximab therapy in children and adults with immune thrombocytopenia. Blood 2012;119:5989-95. [Crossref] [PubMed]
- Serris A, Amoura Z, Canoui-Poitrine F, et al. Efficacy and safety of rituximab for systemic lupus erythematosus-associated immune cytopenias: A multicenter retrospective cohort study of 71 adults. Am J Hematol 2018;93:424-9. [Crossref] [PubMed]
- Rao VK, Oliveira JB. How I treat autoimmune lymphoproliferative syndrome. Blood 2011;118:5741-51. [Crossref] [PubMed]
- Levy R, Mahevas M, Galicier L, et al. Profound symptomatic hypogammaglobulinemia: a rare late complication after rituximab treatment for immune thrombocytopenia. Report of 3 cases and systematic review of the literature. Autoimmun Rev 2014;13:1055-63. [Crossref] [PubMed]
- Einfeld DA, Brown JP, Valentine MA, et al. Molecular cloning of the human B cell CD20 receptor predicts a hydrophobic protein with multiple transmembrane domains. EMBO J 1988;7:711-7. [Crossref] [PubMed]
- Reff ME, Carner K, Chambers KS, et al. Depletion of B cells in vivo by a chimeric mouse human monoclonal antibody to CD20. Blood 1994;83:435-45. [Crossref] [PubMed]
- Administration FaD. Rituxan (Rituximab) Package Insert. 2010.
- Palandri F, Polverelli N, Sollazzo D, et al. Have splenectomy rate and main outcomes of ITP changed after the introduction of new treatments? A monocentric study in the outpatient setting during 35 years. Am J Hematol 2016;91:E267-72. [Crossref] [PubMed]
- Grace RF, Despotovic JM, Bennett CM, et al. Physician decision making in selection of second-line treatments in immune thrombocytopenia in children. Am J Hematol 2018;93:882-8. [Crossref] [PubMed]
- Cuker A, Cines DB, Neunert CE. Controversies in the treatment of immune thrombocytopenia. Curr Opin Hematol 2016;23:479-85. [Crossref] [PubMed]
- Mitsuiki N, Schwab C, Grimbacher B. What did we learn from CTLA-4 insufficiency on the human immune system? Immunol Rev 2019;287:33-49. [Crossref] [PubMed]
- Kuehn HS, Ouyang W, Lo B, et al. Immune dysregulation in human subjects with heterozygous germline mutations in CTLA4. Science 2014;345:1623-7. [Crossref] [PubMed]
- Besnard C, Levy E, Aladjidi N, et al. Pediatric-onset Evans syndrome: Heterogeneous presentation and high frequency of monogenic disorders including LRBA and CTLA4 mutations. Clin Immunol 2018;188:52-7. [Crossref] [PubMed]
- Kostel Bal S, Haskologlu S, Serwas NK, et al. Multiple Presentations of LRBA Deficiency: a Single-Center Experience. J Clin Immunol 2017;37:790-800. [Crossref] [PubMed]
- Gámez-Díaz L, August D, Stepensky P, et al. The extended phenotype of LPS-responsive beige-like anchor protein (LRBA) deficiency. J Allergy Clin Immunol 2016;137:223-30. [Crossref] [PubMed]
- Lopez-Herrera G, Tampella G, Pan-Hammarstrom Q, et al. Deleterious mutations in LRBA are associated with a syndrome of immune deficiency and autoimmunity. Am J Hum Genet 2012;90:986-1001. [Crossref] [PubMed]
- Schubert D, Bode C, Kenefeck R, et al. Autosomal dominant immune dysregulation syndrome in humans with CTLA4 mutations. Nat Med 2014;20:1410-6. [Crossref] [PubMed]
- Verma N, Burns SO, Walker LSK, et al. Immune deficiency and autoimmunity in patients with CTLA-4 (CD152) mutations. Clin Exp Immunol 2017;190:1-7. [Crossref] [PubMed]
- Lee S, Moon JS, Lee CR, et al. Abatacept alleviates severe autoimmune symptoms in a patient carrying a de novo variant in CTLA-4. J Allergy Clin Immunol 2016;137:327-30. [Crossref] [PubMed]
- Grimes AB. Evans Syndrome: Background, Clinical Presentation, Pathophysiology, and Management. In: Despotovic JM, editor. Immune Hematology. Springer International Publishing AG; 2018.
- Coulter TI, Chandra A, Bacon CM, et al. Clinical spectrum and features of activated phosphoinositide 3-kinase delta syndrome: A large patient cohort study. J Allergy Clin Immunol 2017;139:597-606.e4. [Crossref] [PubMed]
- Rao VK, Webster S, Dalm V, et al. Effective "activated PI3Kdelta syndrome"-targeted therapy with the PI3Kdelta inhibitor leniolisib. Blood 2017;130:2307-16. [Crossref] [PubMed]
- Toubiana J, Okada S, Hiller J, et al. Heterozygous STAT1 gain-of-function mutations underlie an unexpectedly broad clinical phenotype. Blood 2016;127:3154-64. [Crossref] [PubMed]
- Bloomfield M, Kanderova V, Parackova Z, et al. Utility of Ruxolitinib in a Child with Chronic Mucocutaneous Candidiasis Caused by a Novel STAT1 Gain-of-Function Mutation. J Clin Immunol 2018;38:589-601. [Crossref] [PubMed]
- Forbes LR, Milner J, Haddad E. Signal transducer and activator of transcription 3: a year in review. Curr Opin Hematol 2016;23:23-7. [Crossref] [PubMed]
- Nabhani S, Schipp C, Miskin H, et al. STAT3 gain-of-function mutations associated with autoimmune lymphoproliferative syndrome like disease deregulate lymphocyte apoptosis and can be targeted by BH3 mimetic compounds. Clin Immunol 2017;181:32-42. [Crossref] [PubMed]
- Pyzik M, Sand KMK, Hubbard JJ, et al. The Neonatal Fc Receptor (FcRn): A Misnomer? Front Immunol 2019;10:1540. [Crossref] [PubMed]
- Newland AC, Sanchez-Gonzalez B, Rejto L, et al. Phase 2 study of efgartigimod, a novel FcRn antagonist, in adult patients with primary immune thrombocytopenia. Am J Hematol 2020;95:178-87. [Crossref] [PubMed]
- Robak T, Kazmierczak M, Jarque I, et al. Phase 2 multiple-dose study of an FcRn inhibitor, rozanolixizumab, in patients with primary immune thrombocytopenia. Blood Adv 2020;4:4136-46. [Crossref] [PubMed]
- Pharmaceuticals D. Dova Pharmaceuticals Announces FDA Approval of Supplemental New Drug Application for DOPTELET® (avatrombopag) for Treatment of Chronic Immune Thrombocytopenia (ITP). 2019. Available online: https://www.globenewswire.com/news-release/2019/06/27/1875237/0/en/Dova-Pharmaceuticals-Announces-FDA-Approval-of-Supplemental-New-Drug-Application-for-DOPTELET-avatrombopag-for-Treatment-of-Chronic-Immune-Thrombocytopenia-ITP.html. Accessed August 20 2020.
- Administration FaD. Doptelet (avatrombopag) Package Insert. 2018.
- Bussel J, Arnold DM, Grossbard E, et al. Fostamatinib for the treatment of adult persistent and chronic immune thrombocytopenia: Results of two phase 3, randomized, placebo-controlled trials. Am J Hematol 2018;93:921-30. [Crossref] [PubMed]
Cite this article as: Kim TO, Despotovic JM. Pediatric immune thrombocytopenia (ITP) treatment. Ann Blood 2021;6:4.