A narrative review of PD-1 and autoimmune diseases
Introduction
Background
Autoimmune diseases comprise a collection of disorders that are characterized by tissue injury resulting from abnormal immune responses to self-autoantigens (1-3). Although the etiology and pathogenesis have not yet been completely elucidated, genetic and environmental factors are generally considered to significantly contribute to the pathogenesis of these autoimmune disorders (2,3). Although autoimmune diseases have been historically considered rare, through rigorous epidemiological studies, they have now been shown to affect at least 3% of the population (4). The incidence and prevalence of autoimmune diseases vary from region to region. For example, multiple sclerosis (MS) is unevenly distributed throughout the world, with a prevalence in tropical regions and in Asia of <5 persons per 100,000, and >200 persons per 100,000 in more temperate regions. Moreover, the incidence of MS in Europe is 0.8–8.7/100,000 person-years, 2.7–7.5/100,000 person-years in North America, and 0.7–3.6/100,000 person-years in Asia and the Middle East (5). Because of the magnitude of the population afflicted with autoimmune diseases, further exploration of factors that contribute to the pathogenesis of these crippling disorders would seem indicated to find more optimal methods of therapy. We present the following article in accordance with the Narrative Review reporting checklist (available at https://aob.amegroups.com/article/view/10.21037/aob-20-86/rc).
Objective
In recent years, the programmed cell death-protein 1 (PD-1, CD279) responsible for regulating tolerance and autoimmunity has been discovered (6-8). There are increasingly appearing reports describing its function and role in autoimmune diseases. This review summarizes current findings and biological functions of PD-1 in several major autoimmune diseases and their potential application to clinical management and future research.
Methods
Information used to write this paper was collected from searches of computerized databases. The main search terms are PD-1 and its related contents, and most of the references come from related studies published in the past 10 years.
Summary of results
PD-1
PD-1, also named CD279, belongs to B7/CD28 immunoglobulin superfamily type I transmembrane glycoprotein. PD-1 is composed of the immunoglobulin-like variable (IgV) domain, the transmembrane domain and the cytoplasmic domain, with a molecular weight in the 50–55 kDa range, which has high sequence homology (about 30%) with CD28, inducible costimulatory molecules (ICOS) and cytotoxic T-lymphocyte-associated protein 4 (CTLA4) (9). Compared with CTLA4, PD-1 is expressed both on the cell membrane and in solution as a monomer, and, due to the lack of proximal cysteine residues on the membrane, homodimerization does not occur (9). Another significant structural feature of PD-1 is the lack of a proline-rich ligand binding motif that binds to the receptors of ICOS, CD28 and CTLA4, which is a must in binding to CD86 and CD80. The different structures for different molecules mean different ligand recognition patterns and signaling mechanisms (9). It is worth noting that the cytoplasmic domain of PD-1 contains two tyrosine signaling motifs: immunotyrosine inhibitory motif (ITIM) and immunotyrosine switch motif (ITSM). ITIM plays a major role in the inhibitory function of PD-1, due to the widely distribution in the structures of immunosuppressive receptors such as killer cell immunoglobulin-like receptor (KIR), CD72 and FcγRIIB (10). Interestingly, Mutation studies using mouse B cell lines have shown that PD-1 is dependent on ITSM to exert its inhibitory effect on T cells (11). The ligand for PD-1 was identified as B7-H1, also named PDL-1, PD-L1 combined with PD-1 can inhibit T cell receptor-mediated lymphocyte proliferation and cytokine secretion (7).
PD-1 is mainly expressed in mature activated T cells and B cells, but also inductively expressed in thymus double-negative CD4-CD8- T cells, natural killer (NK) cells, monocytes, some dendritic cells (DCs), natural killer T cells (NKT) and bone marrow cells. Compared to other CD28 family members, the wider range of expression on T cells of PD-1 suggests broader immune responses regulated by PD-1 (12). Because many types of immune cells including Th1 cell, Th17 cell, follicular helper T cell (Tfh), B cells and T regulatory cells are involved in the pathogenesis of autoimmune diseases (13-18), and since PD-1 is expressed on many types of immune cells, it is necessary to explore the relationship of this cellular diversity between PD-1 and autoimmune diseases. Since PD-1 inhibitor therapy has been widely reported in patients with cancer (2) and several excellent reviews are available (19,20), the role of PD-1 in cancer patients will not be included in this report. PD-1 maintains the balance of the immune system and prevents autoimmunity in a variety of ways, and has been demonstrated in several experimental animal models, including systemic lupus erythematosus (SLE), rheumatoid arthritis (RA), type 1 diabetes (T1D) and MS. This review will describe research progress of PD-1 in these autoimmune diseases.
Research and discovery of PD-1 in RA
RA is an autoimmune disease, which affects about 0.5% of the total population and is defined as an immunologically-mediated inflammatory disorder of the synovium (21-23). Common symptoms of RA include joint swelling and pain caused by synovitis, facet joint involvement with dysfunction, anemia, fatigue, high risk of cardiovascular disease and osteoporosis (10). In recent years, it has been demonstrated that the expression of PD-1 in the synovium of early and established RA is higher than that of normal and OA synovium, while the expression of its ligand programmed death ligand-1 (PD-L1) and PD-L2 is increased at all stages of the progressed RA (arthralgia, undifferentiated arthritis, early RA and established RA) (24). In addition, PD-1 has been found to be expressed in the CD4+ and CD8+ T cells infiltrating the synovium of most patients with RA and the levels of serum soluble PD-1 are increased in early RA patients with positive autoantibodies (24). Since increased sPD-1 levels may interfere with PD-1 signaling leading to defective PD-1 effects on T cells, it is therefore likely that the PD-1 pathway is down-regulated during the clinical progression of RA disease (24). There is a high concentration of sPD-1 in sera and synovial fluids of patients with RA. One study showed that soluble PD-1 aggravates the progression of collagen-induced arthritis through the Th1 and Th17 pathways (25). In a retrospective study, 10 patients who received anti-PD-1 antibody treatment developed arthritis or arthralgia within one month after treatment and were diagnosed with RA or polymyalgia rheumatica (PMR) without a history of rheumatic or autoimmune diseases prior to treatment (26). Some studies have also pointed out that the higher the titer of rheumatoid factor, and other inflammatory markers and the greater the score of disease activity, the higher the expression level of PD-1 on peripheral blood T cells in patients with RA (27). In the synovium of patients with RA, PD-1hiCXCR5-CD4+T cells have been found to increase significantly and are defined by the peripheral helper T cell (TPH) population, which expresses factors that promote B cell development, including Interleukin-21 (IL-21), C-X-C motif chemokine 13 (CXCL13), ICOS and Macrophage activating factor (MAF) (28). At the same time, in pathologically inflamed non-lymphoid tissues, TPH cells seem to be the only cells that can promote B cell response and antibody production (Figure 1) (28). With regard to the finding of PD-1 in RA, some studies have stated that the disease activity score of patients with RA is negatively correlated with the expression of PD-1 on CD4+ and CD8+T cells in peripheral blood (29).
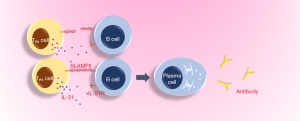
From current research, PD-1 in RA may not play the traditional inhibitory role of this molecule, and further research is required to define its function in different immune cells, and through what mechanism (s) it exerts its impact on RA, through inhibition or promotion.
Research and discovery of PD-1 in SLE
SLE is a systemic autoimmune disease characterized by the production of multiple autoantibodies (30-32). The etiology of SLE involves a multi-factorial set of inflammatory processes, which involve innate and adaptive immune responses, that eventually leads to severe chronic immune disorders. Genetic, epigenetic and environmental factors are thought to play important roles in the pathogenesis of the disorder (33). The expressions of Major histocompatibility complex Class II (MHC-II), inducible T cell costimulatory factor, CD38 and IL-21 in PD-1hiCXCR5-CD4+T cell (TPH) in SLE patients are higher than those in healthy controls, and the relative number of TPH cells in SLE patients has been correlated with the degree of disease (34). Some studies have suggested that the disease is ultimately the result of over-stimulation of the host immune system triggered by repeated exposures to antigens that exceed critical thresholds. In support of this possibility, is the finding in experimental mouse models that overstimulation of mouse CD4 T cells also leads to the development of autoantibody-induced CD4 T cells (AiCD4T), in which the same kinds of autoantibodies are produced and pathological changes seen as in SLE. These pathologic events are accompanied by a significant expansion of a new effector T cell populations, characterized by the expression of programmed death-1 (PD-1) positive, CD27 (low), CD127 (low), CCR7 (low) and CD44 (high) CD62L markers, as well as increased production of IL-2 and IL-6 (35). IL-6 is a crucial pro-inflammatory cytokine that is involved in the pathogenesis and progress of many autoimmune diseases (36-38). In another clinical study, it was also found that the frequency of follicular helper T cells (Tfh, CD4+PD-1+CXCR5+T cells) in peripheral blood of patients with SLE was significantly higher than that of healthy subjects and Tfh cells in peripheral blood could induce B cells to differentiate into plasma cells secreting IgG in vitro. In addition, a positive correlation was observed between the percentage of circulating plasmacytes, serum anti-dsDNA antibodies and antinuclear antibodies. The results of this study also suggest that Tfh cells in peripheral blood may promote the differentiation of autoreactive B cells into plasmablasts that produce autoantibodies, which play a progressive role in the pathogenesis leading eventually to the clinical expression of SLE (39). Interestingly, TPH, Tfh and the new effector T cell group, all share a common characteristic, namely, they all express PD-1 and are closely related to the pathogenesis of SLE. PD-1 gene polymorphism has also been found in SLE. Susceptibility to lupus nephritis and SLE is associated with PD-1.3 and PD-1.5 gene polymorphisms, respectively (40,41). PD-1.6 gene polymorphism may be a protective factor of SLE (42).
Research and discovery of PD-1 in MS
Experimental autoimmune encephalomyelitis (EAE) is another animal model for the study of MS with unknown etiology (43-45), a disorder defined by the destruction of myelin and axons of the central nervous system (CNS) through the invasion of self-reactive T cells that have the capacity to pass through the blood-brain barrier (BBB) (43,46,47). Many cell types in the CNS express PD-1 and its ligands, PD-1 is constitutively expressed in retinal neurons of young mice, while PD-L1 and PD-L2 are found in retinal neurons under inflammatory conditions (48). In the EAE model induced by myelin specific Th17 cell (Th17-EAE) transfer, a large number of Tfh cells were found in the CNS of Th17-EAE mice, and the number of infiltrating Tfh cells was positively correlated with the number of infiltrating B cells at the peak of the disease (49). The frequency of CD4+CXCR5+PD-1+Tfh-like cells are found to be increased in both MS patients and EAE, and Tfh-like cells and B cells are found in the ectopic lymphoid structure of the spinal cord of EAE mice. Tfh-like cells promote antibody production through the interaction between IL-21/IL-21R and CD40L/CD40 and the synergism between Signal transducer and activator of transcription 3 (STAT3) and irregular Nuclear factor kappa-B (NF-κB) signaling pathway in B cells. The adoptive transfer of Tfh-like cells has been shown to aggravate the severity of EAE and delay the remission of EAE (50). Some studies have shown that regulatory T (Treg) cells with high expression of PD-1 in human circulation not only have impaired ability to inhibit CD4+ receptor T cells, but also a high expression of PD-1 characterize a group of dysfunctional Treg cells that produce interferon gamma (51). Indeed, Treg frequency and function intact is important for the prevention of autoimmune diseases (52-54). The level of PD-1 expressed by CD4+CD25brightCD45RA- cells, a subgroup of Treg cells in patients with MS, is significantly higher than that in normal controls. Interestingly, this subgroup includes most of the FoxP3+CD39+ receptor Treg cells, indicating that Treg cells in patients with MS are in a state of exhaustion and dysfunction (55), although others claim that CD39hi Treg represent a superior Treg cell population (30,56).
Research and discovery of PD-1 in T1D
T1D is an autoimmune disease caused by severe destruction of insulin-secreting B-cells in islets (57). Non-obese diabetic (NOD) mice exhibit a spontaneous T1D model with the same genetic and pathophysiological characteristics as human diseases. Some known factors eventually contribute to immune tolerance defects, leading to the development of the disease, including B lymphocytes that produce autoantibodies (58,59), the expansion of autoreactive CD4+ and CD8+ T cells (60), and the activation of the innate immune system (61). It is believed that PD-1 signal plays an important role in the initiation and progression of T1D through multiple checkpoints, studies using in vivo microscopy have shown that T cell receptor (TCR) signals in lymph nodes are truncated during PD-1 ligation (62). PD-1 inhibits germinal center, PD-1 or PD-L1 deficiency in patients with T1D, accompanied by the increase of B cells in germinal center (GC) and the production of insulin autoantibodies (63). In addition, it has been reported that Nivolumab, an anti-PD-1 antibody, can induce fulminant T1D (64). In patients with previous endocrine autoimmunity, there may be more frequent and serious immune-related side effects in anti-PD-1 therapy (65). Autoantibody (AAB) is a marker of T1D mellitus (T1D). Compared with AAB-negative children, newly diagnosed T1D children and children at risk of developing T1D (AAB-positive) had a higher frequency of Treg cells, but less PD-1 expression, and a lower proportion of Forkhead box protein P 3 (FOXP3)+ Treg expressing PD-1 in circulation in AAB-positive high-risk children (66). From the current research, PD-1 tends to be a protective factor in T1D, and T1D may be one of the side effects caused by PD-1 inhibitors in cancer patients (64).
Conclusions
In recent years, PD-1 research has shown significant progress particularly in the field of anti-tumor immunology with significant clinical application. However, the mechanistic role of PD-1 has not been fully clarified, particularly in non-tumor diseases. For example, research findings of the role of PD-1 in autoimmune diseases, does not seem to be consistent. Anti-PD-1 therapy for tumor patients may enhance side effects of autoimmune diseases, such as T1D. When PD-1 is blocked, some side effects of autoimmune disease are seen as an expression of an adverse immunosuppressive effect. However, increased expression of PD-1 has also been observed in autoimmune diseases, such as RA, SLE, and is related to disease activity. In a recent report, injection of immunotoxin targeting PD-1 expression into autoimmune diabetic mice cells was found to delay the onset of the disease, and injection of immunotoxin into paralyzed mice with EAE was also shown to relieve symptoms (67). Thus, it is likely that cells expressing PD-1 may paradoxically also promote the disease in autoimmune diseases, which is different from the traditional inhibitory role attributed to PD-1. Therefore, the role of PD-1 in autoimmune diseases is quite promising, warrants a further in depth research.
Acknowledgments
Funding: None.
Footnote
Reporting Checklist: The authors have completed the Narrative Review reporting checklist. Available at https://aob.amegroups.com/article/view/10.21037/aob-20-86/rc
Conflicts of Interest: All authors have completed the ICMJE uniform disclosure form (available at https://aob.amegroups.com/article/view/10.21037/aob-20-86/coif). The authors have no conflicts of interest to declare.
Ethical Statement:
Open Access Statement: This is an Open Access article distributed in accordance with the Creative Commons Attribution-NonCommercial-NoDerivs 4.0 International License (CC BY-NC-ND 4.0), which permits the non-commercial replication and distribution of the article with the strict proviso that no changes or edits are made and the original work is properly cited (including links to both the formal publication through the relevant DOI and the license). See: https://creativecommons.org/licenses/by-nc-nd/4.0/.
References
- Bi X, Guo XH, Mo BY, et al. LncRNA PICSAR promotes cell proliferation, migration and invasion of fibroblast-like synoviocytes by sponging miRNA-4701-5p in rheumatoid arthritis. EBioMedicine 2019;50:408-20. [Crossref] [PubMed]
- Liu Y, Pan YF, Xue YQ, et al. uPAR promotes tumor-like biologic behaviors of fibroblast-like synoviocytes through PI3K/Akt signaling pathway in patients with rheumatoid arthritis. Cell Mol Immunol 2018;15:171-81. [Crossref] [PubMed]
- Zou Y, Xu S, Xiao Y, et al. Long noncoding RNA LERFS negatively regulates rheumatoid synovial aggression and proliferation. J Clin Invest 2018;128:4510-24. [Crossref] [PubMed]
- Wang L, Wang FS, Gershwin ME. Human autoimmune diseases: a comprehensive update. J Intern Med 2015;278:369-95. [Crossref] [PubMed]
- Milo R, Kahana E. Multiple sclerosis: geoepidemiology, genetics and the environment. Autoimmun Rev 2010;9:A387-94. [Crossref] [PubMed]
- Curran C S, Gupta S, Sanz I, et al. PD-1 immunobiology in systemic lupus erythematosus. J Autoimmun 2019;97:1-9. [Crossref] [PubMed]
- Freeman GJ, Long AJ, Iwai Y, et al. Engagement of the PD-1 immunoinhibitory receptor by a novel B7 family member leads to negative regulation of lymphocyte activation. J Exp Med 2000;192:1027-34. [Crossref] [PubMed]
- Ishida Y, Agata Y, Shibahara K, et al. Induced expression of PD-1, a novel member of the immunoglobulin gene superfamily, upon programmed cell death. EMBO J 1992;11:3887-95. [Crossref] [PubMed]
- Saresella M, Rainone V, Al-Daghri NM, et al. The PD-1/PD-L1 pathway in human pathology. Curr Mol Med 2012;12:259-67. [Crossref] [PubMed]
- Zamani MR, Aslani S, Salmaninejad A, et al. PD-1/PD-L and autoimmunity: A growing relationship. Cell Immunol 2016;310:27-41. [Crossref] [PubMed]
- Chemnitz JM, Parry RV, Nichols KE, et al. SHP-1 and SHP-2 associate with immunoreceptor tyrosine-based switch motif of programmed death 1 upon primary human T cell stimulation, but only receptor ligation prevents T cell activation. J Immunol 2004;173:945-54. [Crossref] [PubMed]
- Chen L, Flies D B. Molecular mechanisms of T cell co-stimulation and co-inhibition. Nat Rev Immunol 2013;13:227-42. [Crossref] [PubMed]
- Zhou L, Wang J, Li J, et al. 1, 25-Dihydroxyvitamin D3 Ameliorates Collagen-Induced Arthritis via Suppression of Th17 Cells Through miR-124 Mediated Inhibition of IL-6 Signaling. Front Immunol 2019;10:178. [Crossref] [PubMed]
- Yang S, Xie C, Chen Y, et al. Differential roles of TNFalpha-TNFR1 and TNFalpha-TNFR2 in the differentiation and function of CD4(+)Foxp3(+) induced Treg cells in vitro and in vivo periphery in autoimmune diseases. Cell Death Dis 2019;10:27. [Crossref] [PubMed]
- Liu Y, Lan Q, Lu L, et al. Phenotypic and functional characteristic of a newly identified CD8+ Foxp3- CD103+ regulatory T cells. J Mol Cell Biol 2014;6:81-92. [Crossref] [PubMed]
- Li N, Wang JC, Liang TH, et al. Pathologic finding of increased expression of interleukin-17 in the synovial tissue of rheumatoid arthritis patients. Int J Clin Exp Pathol 2013;6:1375-9. [PubMed]
- Chen M, Lin X, Liu Y, et al. The function of BAFF on T helper cells in autoimmunity. Cytokine Growth Factor Rev 2014;25:301-5. [Crossref] [PubMed]
- Zheng S G, Wang J, Horwitz D A. Cutting edge: Foxp3+CD4+CD25+ regulatory T cells induced by IL-2 and TGF-beta are resistant to Th17 conversion by IL-6. J Immunol 2008;180:7112-6. [Crossref] [PubMed]
- Zou W, Wolchok J D, Chen L. PD-L1 (B7-H1) and PD-1 pathway blockade for cancer therapy: Mechanisms, response biomarkers, and combinations. Sci Transl Med 2016;8:328rv4. [Crossref] [PubMed]
- Yang B, Liu T, Qu Y, et al. Progresses and Perspectives of Anti-PD-1/PD-L1 Antibody Therapy in Head and Neck Cancers. Front Oncol 2018;8:563. [Crossref] [PubMed]
- Yang M, Liu Y, Mo B, et al. Helios but not CD226, TIGIT and Foxp3 is a Potential Marker for CD4(+) Treg Cells in Patients with Rheumatoid Arthritis. Cell Physiol Biochem 2019;52:1178-92. [Crossref] [PubMed]
- Liu F, Feng X X, Zhu S L, et al. Sonic Hedgehog Signaling Pathway Mediates Proliferation and Migration of Fibroblast-Like Synoviocytes in Rheumatoid Arthritis via MAPK/ERK Signaling Pathway. Front Immunol 2018;9:2847. [Crossref] [PubMed]
- Zhu S L, Huang JL, Peng WX, et al. Inhibition of smoothened decreases proliferation of synoviocytes in rheumatoid arthritis. Cell Mol Immunol 2017;14:214-22. [Crossref] [PubMed]
- Guo Y, Walsh AM, Canavan M, et al. Immune checkpoint inhibitor PD-1 pathway is down-regulated in synovium at various stages of rheumatoid arthritis disease progression. PLoS One 2018;13:e0192704. [Crossref] [PubMed]
- Liu C, Jiang J, Gao L, et al. Soluble PD-1 aggravates progression of collagen-induced arthritis through Th1 and Th17 pathways. Arthritis Res Ther 2015;17:340. [Crossref] [PubMed]
- Belkhir R, Burel S L, Dunogeant L, et al. Rheumatoid arthritis and polymyalgia rheumatica occurring after immune checkpoint inhibitor treatment. Ann Rheum Dis 2017;76:1747-50. [Crossref] [PubMed]
- Luo Q, Ye J, Zeng L, et al. Elevated expression of PD1 on T cells correlates with disease activity in rheumatoid arthritis. Mol Med Rep 2018;17:3297-305. [PubMed]
- Rao DA, Gurish MF, Marshall JL, et al. Pathologically expanded peripheral T helper cell subset drives B cells in rheumatoid arthritis. Nature 2017;542:110-4. [Crossref] [PubMed]
- Li S, Liao W, Chen M, et al. Expression of programmed death-1 (PD-1) on CD4+ and CD8+ T cells in rheumatoid arthritis. Inflammation 2014;37:116-21. [Crossref] [PubMed]
- Zhang X, Ouyang X, Xu Z, et al. CD8+CD103+ iTregs Inhibit Chronic Graft-versus-Host Disease with Lupus Nephritis by the Increased Expression of CD39. Mol Ther 2019;27:1963-73. [Crossref] [PubMed]
- Xiao ZX, Olsen N, Zheng SG. The essential role of costimulatory molecules in systemic lupus erythematosus. Lupus 2019;28:575-82. [Crossref] [PubMed]
- Huang Z, Fu B, Zheng SG, et al. Involvement of CD226+ NK cells in immunopathogenesis of systemic lupus erythematosus. J Immunol 2011;186:3421-31. [Crossref] [PubMed]
- Mills JA. Systemic lupus erythematosus. N Engl J Med 1994;330:1871-9. [Crossref] [PubMed]
- Lin J, Yu Y, Ma J, et al. PD-1+CXCR5-CD4+T cells are correlated with the severity of systemic lupus erythematosus. Rheumatology (Oxford) 2019;58:2188-92. [Crossref] [PubMed]
- Miyazaki Y, Tsumiyama K, Yamane T, et al. Expansion of PD-1-positive effector CD4 T cells in an experimental model of SLE: contribution to the self-organized criticality theory. Kobe J Med Sci 2013;59:E64-71. [PubMed]
- Chen X, Su W, Wan T, et al. Sodium butyrate regulates Th17/Treg cell balance to ameliorate uveitis via the Nrf2/HO-1 pathway. Biochem Pharmacol 2017;142:111-9. [Crossref] [PubMed]
- Peng YQ, Qin ZL, Fang SB, et al. Effects of myeloid and plasmacytoid dendritic cells on ILC2s in patients with allergic rhinitis. J Allergy Clin Immunol 2020;145:855-67.e8. [Crossref] [PubMed]
- Zhang M, Zhang L, Li H, et al. Circulating T follicular helper cells are associated with rapid virological response in chronic hepatitis C patients undergoing peginterferon therapy. Int Immunopharmacol 2016;34:235-43. [Crossref] [PubMed]
- Zhang X, Lindwall E, Gauthier C, et al. Circulating CXCR5+CD4+helper T cells in systemic lupus erythematosus patients share phenotypic properties with germinal center follicular helper T cells and promote antibody production. Lupus 2015;24:909-17. [Crossref] [PubMed]
- Lee YH, Woo JH, Choi SJ, et al. Association of programmed cell death 1 polymorphisms and systemic lupus erythematosus: a meta-analysis. Lupus 2009;18:9-15. [Crossref] [PubMed]
- Prokunina L, Castillejo-Lopez C, Oberg F, et al. A regulatory polymorphism in PDCD1 is associated with susceptibility to systemic lupus erythematosus in humans. Nat Genet 2002;32:666-9. [Crossref] [PubMed]
- Gao J, Gai N, Wang L, et al. Meta-analysis of programmed cell death 1 polymorphisms with systemic lupus erythematosus risk. Oncotarget 2017;8:36885-97. [Crossref] [PubMed]
- Li H, Deng Y, Liang J, et al. Mesenchymal stromal cells attenuate multiple sclerosis via IDO-dependent increasing the suppressive proportion of CD5+ IL-10+ B cells. Am J Transl Res 2019;11:5673-88. [PubMed]
- Zhou X, Xia Z, Lan Q, et al. BAFF promotes Th17 cells and aggravates experimental autoimmune encephalomyelitis. PLoS One 2011;6:e23629. [Crossref] [PubMed]
- Owens T, Sriram S. The immunology of multiple sclerosis and its animal model, experimental allergic encephalomyelitis. Neurol Clin 1995;13:51-73. [Crossref] [PubMed]
- Viglietta V, Baecher-Allan C, Weiner HL, et al. Loss of functional suppression by CD4+CD25+ regulatory T cells in patients with multiple sclerosis. J Exp Med 2004;199:971-79. [Crossref] [PubMed]
- Gao Y, Tang J, Chen W, et al. Inflammation negatively regulates FOXP3 and regulatory T-cell function via DBC1. Proc Natl Acad Sci U S A 2015;112:E3246-54. [Crossref] [PubMed]
- Chen L, Pai V, Levinson R, et al. Constitutive neuronal expression of the immune regulator, programmed death 1 (PD-1), identified during experimental autoimmune uveitis. Ocul Immunol Inflamm 2009;17:47-55. [Crossref] [PubMed]
- Quinn JL, Kumar G, Agasing A, et al. Role of TFH Cells in Promoting T Helper 17-Induced Neuroinflammation. Front Immunol 2018;9:382. [Crossref] [PubMed]
- Guo J, Zhao C, Wu F, et al. T Follicular Helper-Like Cells Are Involved in the Pathogenesis of Experimental Autoimmune Encephalomyelitis. Front Immunol 2018;9:944. [Crossref] [PubMed]
- Lowther DE, Goods BA, Lucca LE, et al. PD-1 marks dysfunctional regulatory T cells in malignant gliomas. JCI Insight 2016;1:e85935. [Crossref] [PubMed]
- Su W, Fan H, Chen M, et al. Induced CD4+ forkhead box protein-positive T cells inhibit mast cell function and established contact hypersensitivity through TGF-beta1. J Allergy Clin Immunol 2012;130:444-52.e7. [Crossref] [PubMed]
- Yang S, Wang J, Brand DD, et al. Role of TNF-TNF Receptor 2 Signal in Regulatory T Cells and Its Therapeutic Implications. Front Immunol 2018;9:784. [Crossref] [PubMed]
- Zheng SG, Wang JH, Gray JD, et al. Natural and induced CD4+CD25+ cells educate CD4+CD25- cells to develop suppressive activity: the role of IL-2, TGF-beta, and IL-10. J Immunol 2004;172:5213-21. [Crossref] [PubMed]
- Sambucci M, Gargano F, De Rosa V, et al. FoxP3 isoforms and PD-1 expression by T regulatory cells in multiple sclerosis. Sci Rep 2018;8:3674. [Crossref] [PubMed]
- Gu J, Ni X, Pan X, et al. Human CD39(hi) regulatory T cells present stronger stability and function under inflammatory conditions. Cell Mol Immunol 2017;14:521-8. [Crossref] [PubMed]
- Atkinson MA. The pathogenesis and natural history of type 1 diabetes. Cold Spring Harb Perspect Med 2012;2:a007641. [Crossref] [PubMed]
- Xu A, Liu Y, Chen W, et al. TGF-beta-Induced Regulatory T Cells Directly Suppress B Cell Responses through a Noncytotoxic Mechanism. J Immunol 2016;196:3631-41. [Crossref] [PubMed]
- Zhong H, Liu Y, Xu Z, et al. TGF-beta-Induced CD8(+)CD103(+) Regulatory T Cells Show Potent Therapeutic Effect on Chronic Graft-versus-Host Disease Lupus by Suppressing B Cells. Front Immunol 2018;9:35. [Crossref] [PubMed]
- Ye C, Brand D, Zheng S G. Targeting IL-2: an unexpected effect in treating immunological diseases. Signal Transduct Target Ther 2018;3:2. [Crossref] [PubMed]
- Zhang W, Zhou L, Dang J, et al. Human Gingiva-Derived Mesenchymal Stem Cells Ameliorate Streptozoticin-induced T1DM in mice via Suppression of T effector cells and Up-regulating Treg Subsets. Sci Rep 2017;7:15249. [Crossref] [PubMed]
- Fife BT, Pauken KE, Eagar TN, et al. Interactions between PD-1 and PD-L1 promote tolerance by blocking the TCR-induced stop signal. Nat Immunol 2009;10:1185-92. [Crossref] [PubMed]
- Martinov T, Swanson LA, Breed ER, et al. Programmed Death-1 Restrains the Germinal Center in Type 1 Diabetes. J Immunol 2019;203:844-52. [Crossref] [PubMed]
- Miyoshi Y, Ogawa O, Oyama Y. Nivolumab, an Anti-Programmed Cell Death-1 Antibody, Induces Fulminant Type 1 Diabetes. Tohoku J Exp Med 2016;239:155-8. [Crossref] [PubMed]
- Akturk HK, Alkanani A, Zhao Z, et al. PD-1 Inhibitor Immune-Related Adverse Events in Patients With Preexisting Endocrine Autoimmunity. J Clin Endocrinol Metab 2018;103:3589-92. [Crossref] [PubMed]
- Vecchione A, Di Fonte R, Gerosa J, et al. Reduced PD-1 expression on circulating follicular and conventional FOXP3(+) Treg cells in children with new onset type 1 diabetes and autoantibody-positive at-risk children. Clin Immunol 2020;211:108319. [Crossref] [PubMed]
- Zhao P, Wang P, Dong S, et al. Depletion of PD-1-positive cells ameliorates autoimmune disease. Nat Biomed Eng 2019;3:292-305. [Crossref] [PubMed]
Cite this article as: Deng Y, Huang F, Wang J. A narrative review of PD-1 and autoimmune diseases. Ann Blood 2022;7:31.